Products including thermal paste and compound for coating on Rugged Industrial Computers/Comms , SSD, and 5G & AIoT devices provide industry lowest thermal resistance. The material is designed to be used like a traditional thermal interface material between heat source and heat sink or other heat transfer device or metal chassis.
Graphene heatsinks and thermal interface materials are innovative solutions that have revolutionized the field of thermal management. When used as a heatsink material, it efficiently dissipates heat away from electronic components, ensuring their optimal performance and longevity. Additionally, graphene-based thermal interface materials act as efficient bridges between the heat source and the heatsink, enhancing heat transfer and minimizing thermal resistance. These materials exhibit excellent stability, durability, and compatibility with various substrates, making them ideal for a wide range of applications in industries such as electronics, aerospace, and automotive. With their exceptional thermal management capabilities, graphene heatsinks and thermal interface materials pave the way for more efficient and reliable electronic devices in the future.
Developing a novel highly thermal conductive and light-weight graphene heatsink. Composed by vertically-aligned and continuous graphene structures, heat transport was highly efficient from the base to fin structures inside the heatsink The maximum through-plane thermal conductivity of graphene heatsink can be up to 1000~1500 W/mK, which is over 7 times higher than aluminum, and even outperforms copper about 4 times. Graphene heatsink demonstrated outstanding cooling performance which was superior to copper heatsink with the same dimension and same power input. Noticeably, the graphene heatsink also has important advantages of light-weight and high emissivity. The measured density (1.1 g/cm 3 ) is only one-eighth of copper and less than half of aluminum and emissivity is about ten times higher than pure copper and aluminum. The resulting graphene heatsink thus opens new opportunities for addressing large heat dissipation issues in weight driven electronics and other high power systems.
TECHNOLOGY
Large-area Ultrathin Graphene Improves Thermal Conductivity
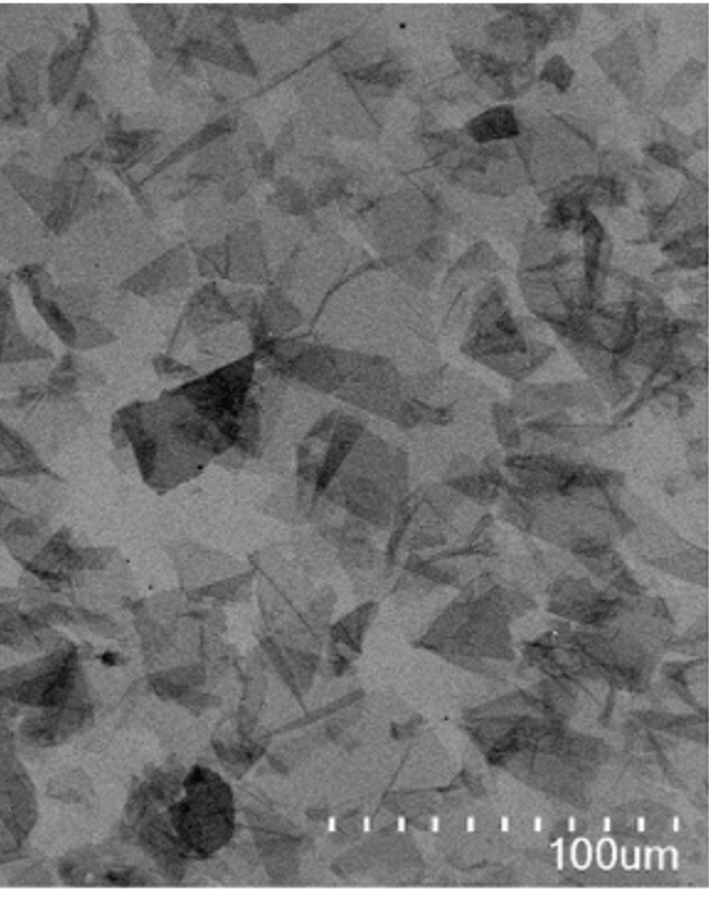
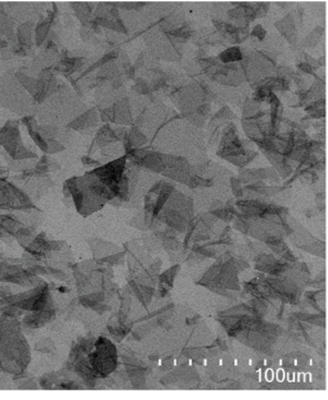
TiKOUS is a global leader in natural cooling with industrial-grade graphene
The thermal conductivity of graphene is depending on the diffusive and ballistic conditions at higher and lower temperature ranges respectively. The better thermal conductivity of graphene materials is highly dependent on the quality of graphene sheets.
From a chemical reaction point of view, the pure form of graphene is mostly not reactive. The chemical properties of graphene are critically influenced by its surface characteristics and the thickness of graphene layers. Single-layer graphene materials are more highly chemically reactive than multi-layer graphene materials.
Unlike traditional graphene, our products are developed and manufactured on large scale with high-quality standards to prevent the inconsistency and batch-dependency of the graphene.
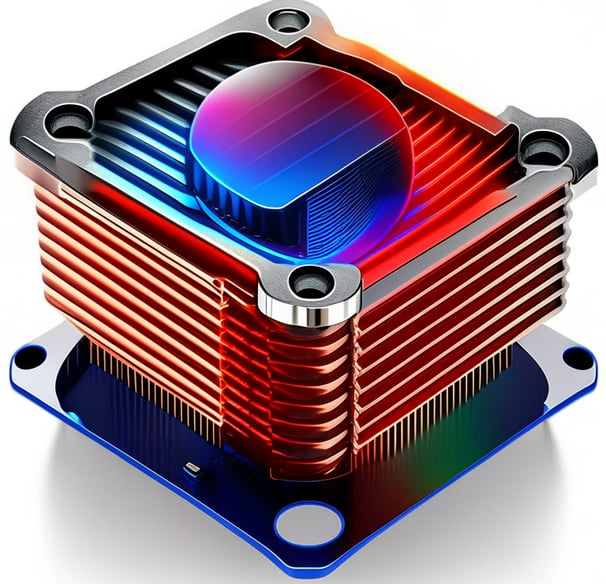
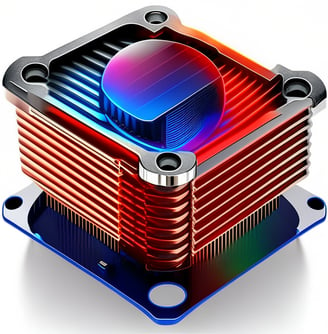
Copyright © 2024 TiKOUS, Inc. All rights reserved.